Keeping cool beyond ice
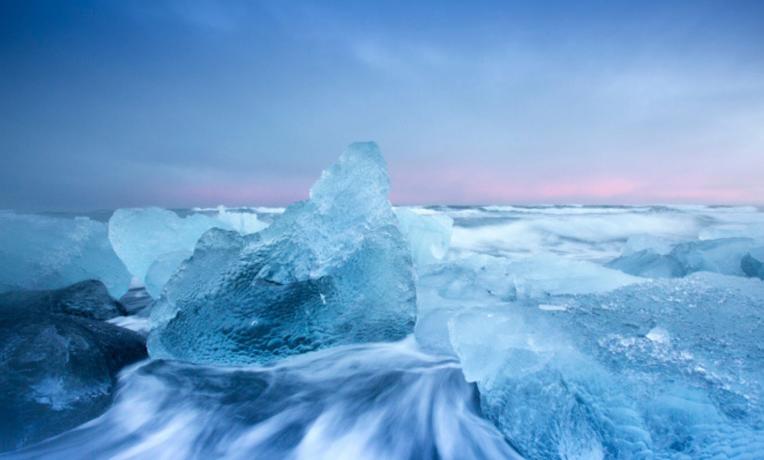
By Inge Ruigrok
Gibson’s research at the Manchester Institute of Biotechnology draws inspiration from nature’s ultimate survivors-extremophiles like Arctic fish, which thrive in extreme cold. ‘If you or I were to swim in the Arctic, we wouldn’t survive for long, but the waters are full of fish,´ he says. ‘The secret is in how these cold-blooded creatures produce what we call antifreeze proteins. We wondered: can we create molecules or materials that mimic these proteins to help stabilise sensitive medicines, like biologics?’
Biologics are protein-based therapies like insulin and antibody treatments, which are commonly used for autoimmune diseases and cancer care. They also include engineered cells-human cells taken from a patient’s body, modified, and reintroduced to treat conditions like blood cancers.
One of the biggest challenges with biologics, especially with living cells, is the cold chain—keeping these cells fresh as they are transported to the patient. Gibson: ‘You need to maximise the number of living cells that survive when they’re taken out of the freezer. Yet freezing is a complex, sometimes unpredictable process, and when cells are thawed, they can sometimes 'wake up' with less energy—like feeling sluggish on a Monday morning.’
Ice crystals
The challenge then is to make cells consistently more active after thawing to improve their effectiveness as therapies. For this, it is necessary to control ice formation. Gibson and his team have discovered new polymers, which are large molecules that attach to ice crystals and stop them from growing. ‘We’re also working to reduce the amount of conventional cryoprotectants-antifreeze-like substances used to protect cells during freezing’, he says.’ Since these compounds enter the body, it's essential to minimise their use or find safer alternatives.’
Developing molecules and materials that can safely store cells, tissues, and proteins takes time. Gibson: ‘Usually, my team comes up with an idea, we make the material, test it, and most of the time, it doesn’t work. The failure rate is high, and progress can be slow.’ Besides, one of the biggest challenges in pharmaceuticals is the long process of safety testing, which often causes delays.
To speed up the process, Gibson turned to machine learning with computational scientist Gabriele Sosso at the University of Warwick. ‘We train software with large datasets to find patterns that humans might miss,’ he explains. ‘The goal is to teach the system to analyse both successful and unsuccessful experiments and predict which molecules might work better. We focused on compounds that have already been checked for toxicity and synthesis, so we knew about their safety and properties upfront, which saves time.
Remarkably, the model flagged several compounds that worked exceptionally well, even though they looked nothing like traditional antifreeze proteins. These molecules attach to ice crystals and prevent them from growing. In our tests, adding these compounds to blood allowed us to reduce the amount of antifreeze needed, showing great potential for improving cryopreservation.
This has dramatically accelerated our progress. Over my career, since starting in 2010, we’ve discovered around 5-10 useful molecules. Using the machine learning model, we identified 10-12 promising candidates in just a single day.’
Room temperature
During the COVID-19 pandemic, the need for practical solutions became evident, as mRNA and some protein-based vaccines required cold storage, making distribution challenging in regions with limited infrastructure. ‘Typically, to freeze and store cells and tissues, we use temperatures around -80°C because colder conditions improve their survival,’ Gibson explains. ‘Generating and maintaining extreme cold needs a lot of electricity, which isn’t feasible in many parts of the world.’
Gibson’s team is developing storage methods that can withstand warmer conditions, such as -20°C (the temperature of a domestic freezer) or even room temperature. ‘This is much easier to sustain with solar panels or even a car engine for power during transport,’ he adds. ‘We’re also investigating the possibility of storing certain treatments at room temperature. While room temperature varies across regions, the key idea is to stabilise these materials without relying on active refrigeration.’
Shaking proteins
One way to store proteins without freezing them is in a gel, which also helps prevent aggregation. ‘For example, insulin is sensitive to how it’s handled. If it’s shaken too vigorously, the proteins can clump together, which is problematic because aggregation can reduce their effectiveness or even make them toxic,’ Gibson explains.
However, storing proteins in a gel creates another problem: how to release the protein so it can be given to patients. Gibson explains, ‘Typically, we need an additional chemical, like sugar, to help with the release. Unfortunately, this process can take about an hour, complicating the treatment. In traditional methods, proteins are often released slowly from the gel, and all the gel components remain mixed in. This is difficult because converting a protein from gel to liquid for injection makes it hard to predict how the gel components will interact.’
Gibson’s team worked with material chemist Dave Adams at Glasgow University to develop a gel that can be stored inside a standard squeezable syringe without refrigeration. The syringe is designed with a filter that allows only the active drug to be injected, leaving the gel behind. ‘When you push down on the syringe, the force temporarily breaks the gel. This means that instead of using a chemical trigger for release, all you need to do is press down on the syringe plunger’, Gibson explains. ‘Another clever feature is the filter at the end of the syringe. As the protein is expelled, the filter captures all the gel components, ensuring that only pure protein mixed with water or a saline solution is injected.
Our tests showed that the proteins remained stable without refrigeration, even under extreme conditions. We subjected the gels to high-intensity shaking and even threw them against walls and on the floor to test their durability. The results were promising: the gels maintained their structure and effectively protected the proteins, showing their ability to withstand the handling typically encountered during transportation.’
Freezing water
Besides storage without freezing, Gibson and his team are also investigating how to control the freezing process itself. This involved finding effective ways to induce ice formation, and once again, the team turned to nature for inspiration.
‘When we're freezing cells, the water doesn't turn to ice at 0°C; it freezes at temperatures between -5°C and -20°C’, Gibson explains. ‘This is due to both the cryoprotectants we're adding, and a phenomenon called supercooling. Water takes a while to freeze, which creates variability in results. For instance, under the same conditions, one time the water might freeze at -5°C, another time at -10°C, and yet another at -15°C. Even with everything being identical-same samples, same freezer, and the same time-the outcomes might differ. This variability in freezing can lead to inconsistent outcomes, which is problematic in scientific and medical contexts.’
Through a collaboration with Kew Royal Botanic Gardens in London and Thomas Whale at Leeds University, Gibson is exploring methods that ensure ice forms consistently at specific temperatures. ‘It might sound strange, but we identified components from pollen that help plants reproduce, and when we wash and extract it, it promotes ice formation. We are also investigating the roles of ice-binding proteins, which can help control ice crystal formation during freezing. Additionally, certain bacteria produce ice, and we’re exploring the possibility of incorporating components of these into our research because they can be beneficial. I'm excited about this unexpected direction we've taken, addressing the fundamental question of how we can make ice form at higher temperatures. ‘
In addition to biomedical applications, Gibson believes that this research can have broader implications for engineering and infrastructure. ‘Without freezing and cooling technologies, many essential services—like food storage and air travel—would be compromised. For example, planes can't take off in winter if there’s ice buildup, a problem increasingly complicated by climate change and more frequent frost-related issues. By understanding how life survives in extreme conditions, we can develop new solutions for space exploration and climate resilience. This requires us to address not only high temperatures but also the complexities brought by climate variability.’
Biography
Professor Matthew Gibson holds a Chair in Sustainable Biomaterials at the University of Manchester, UK. Matt was a Royal Society Industry Fellow with Cytiva (2019-2023), and has held an ERC Starter (2015-2020) and Consolidator Grant (2020 – present) and is co-founder of the biotechnology spin-out Cryologyx Ltd. Matt has been awarded several prizes including the Corday-Morgan, McBain, Dextra and MacroGroup Young Researcher’s medals as well prizes from the American Chemical Society, and an RSC Horizon Prize for ‘Team Ice’. Professor Gibson’s multidisciplinary research group focusses on developing new materials to address challenges in Biotechnology and Healthcare with a particular focus on cryobiology.